Grade Level
All
minutes
1-3 hours
subject
Physical Science
stem practices
Planning and Carrying Out Investigations
Activity Type:
Engineering design challenge, experiment, solar system, STEAM, Family activity, space
The Sun is the star at the center of our solar system. Eight planets travel in orbits around our nearest star, including our home, the Earth. Many planets, like our own, have moons circling them. There are dwarf planets like Pluto, Ceres, and Eris hidden among the Asteroid Belt and at the very edges of the solar system near the Kuiper Belt, which is home to the most ancient asteroids. Even further beyond that, there is the mysterious Oort Cloud, a collection of icy objects that gives birth to dazzling comets with long tails, vaporized by the heat of the Sun. And last but certainly not least, there are loads of random “space rocks” and meteoroids floating through the solar system, sometimes falling towards Earth as “shooting stars” or crashing into moons or planets and leaving craters.
All of these objects in our solar system are in motion, creating a massive, complex dance around the Sun, which is in motion itself. But what started that movement and what keeps it going? Why do planets all travel in elliptical orbits around the sun? Why do the planets orbit the Sun at all? And while we’re at it, why are all the planets in our solar system round? There must be a reason!
Can’t wait to get started? Jump to the experiment!
Gravity In Our Solar System
All of the objects in our solar system are acted upon by forces. Forces are pushes or pulls. Every force has both a strength and a direction. Applying a force on something–like pushing a toy car–can start or stop the motion of that object. The stronger the push or pull, the bigger the force. When things run into one another, they apply force to one another, and their direction and speed can change as a result–like when a player hits a baseball with a bat.
Our solar system formed 4.6 billion years ago when a nearby star exploded, sending waves of energy out into space. When hit by a wave, one big cloud of stellar dust and gas, called a nebula, collapsed into a spinning disk. That disk pulled more dust and gases into the center due to a force called gravity. Eventually, the pressure at the core of the disk was so strong that the materials fused together, releasing tremendous energy. Our star, the Sun, was born!
Download the free poster associated with the video.
Nearly all of the matter from the original cloud of dust was pulled into the Sun, the biggest, most massive object in our solar system. Eventually, the leftover bits of material like rocks and ice collided and began to clump together, drawn by gravitational pull between them. Slowly these became the planets. Some newly formed planets had a large enough mass, and with it, a large gravitational force, to pull on and capture smaller objects that were moving past them. These smaller objects fell into orbit around larger planets, becoming their moons.
As the planets began to settle into orbits the pull of gravity from their own center of mass pulled them in on themselves, tugging at each planet’s mass equally towards its center. This strong internal gravitational pull gives planets their round, spherical shape.
But that’s not the only force working on planets. The inertia of that early explosion that set everything in our solar system in motion is still acting on the planets today.
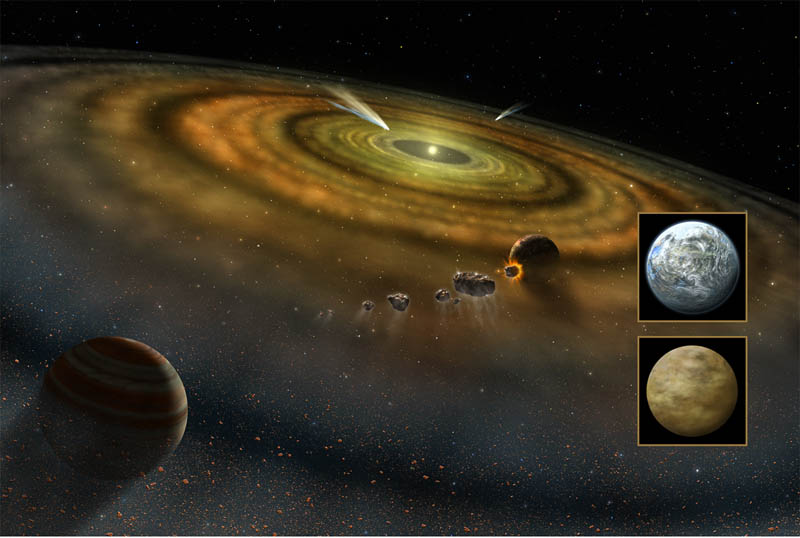
An object in motion will remain in motion at the same speed and going in the same direction unless some other force acts on it. For example, when you’re playing soccer and kick a ball, you change its direction on impact. But in space, once all that matter collected into planets and moons, there wasn’t a whole lot for them to knock into anymore. There’s a lot of space in space.
Those early planets may have just kept flying along, but the incredibly strong pull of gravity from that new star, the Sun, was at work as well. Because the Sun is the largest, most massive object in our solar system, it also has the strongest gravitational force in our solar system. It pulls every one of the planets (and everything else) toward its center of mass. Essentially there was a tug-of-war between the inertia of the planets and the gravitational force of the Sun. Those unbalanced forces pulled the planets in two directions at once, ultimately resulting in a circular force. When that happens we call it a centripetal force—a force that keeps objects moving in a circular path. That circular motion is the reason for the planets’ orbit around the Sun.
Today you’re going to experiment with forces like those that set our solar system in motion. So gather your materials and get ready to explore the forces of our solar system!
Materials
2 plastic split eggs (or other small reclosable plastic containers)
Glass marbles or other small balls (or make some from clay)
Tape or stickers to hold the eggs closed
Dressmakers’ tape measure or meter stick
Embroidery floss
Small disposable plate with a rim
Scissors
Scale (optional)
Two pans, bins, or baskets to catch the eggs (Tip: Use bins made of different materials so that the eggs make different sounds when they land. Test each egg alone a few times so you recognize the sound.)
Safety and clean-up: Some of these experiments are best performed outdoors or in a large, unobstructed space. Before performing any of the activities ensure that you have an appropriate amount of space and that no one will be hit by moving objects. Take care with the glass marbles, as they can break if used too roughly or dropped on a hard floor. You may want to consider eye protection.
A Gravity, Mass, and Height Experiment
Just as the Sun’s gravity pulls on the Earth in the direction of the center of the solar system, the Earth’s gravity pulls on you towards its center of mass. Every object on Earth is pulled toward Earth’s center. But guess what? You’re pulling on the Earth! That’s right, you’re pulling the Earth toward your center of gravity too. However, Earth has much, much more mass than you do, so Earth’s gravity is much, much greater than yours. More mass, more gravity.
You may be thinking, “If that’s the case, why isn’t the Sun pulling me off the Earth? After all, the Sun has much more mass than the Earth, right?” That’s true, but the Sun is also very far away. The distance between two objects affects the strength of gravitational force. You are closer to the Earth so the force of Earth’s gravity on you is much stronger. It doesn’t mean the Sun isn’t pulling on you too, you just don’t feel it as strongly.
In the next two experiments, you will perform drop tests on two objects—plastic eggs—that have the same shape and size, and compare how quickly they fall to the Earth. It is important that you control, or keep the same, the size of the plastic eggs that you are dropping in your experiments so that you can be sure that things like air resistance aren’t affecting your results.
First, you will vary the mass of your eggs by adding marbles to one of them. Then you will vary the height from which they are dropped. In each case, you will compare how quickly the two eggs hit the ground.
Before you start, read over the activities below. Make a prediction about what you think will happen with each drop experiment.
Set Up
- Attach your tape measure, yardstick, or meterstick to a wall so that that starting point is against the floor. Alternatively, you can place marks in pencil on the wall every 6 inches from the floor to 3 feet or more above the floor.
- Place a bin at the bottom of the tape measure or ruler to catch your eggs. (Tip: Use two different bins–one for each egg–made of different materials so that the eggs make different sounds when they land. Test a few times with each egg alone so that you recognize the sound.)
- If desired, prepare a smartphone or camera with a slow-motion video feature to film the egg drops in slow motion.
- When assembling the eggs, use tape or stickers to hold the eggs closed. If desired, measure the weight of each egg and record it.
Activity 1: Drop Objects From Different Heights
- Assemble both plastic eggs. Add 4 marbles to each egg.
- With the pointy end facing up, hold one egg at 3 feet above the floor and the other egg at 2 ½ feet above the floor.
- Count to three and drop the eggs at the same time. Listen and watch carefully to see when it hits the floor. Record your results.
- Repeat the experiment several times.
- Vary the height you drop the two eggs from, increasing the drop distance for one egg by 6 inches each time. Record your observations.
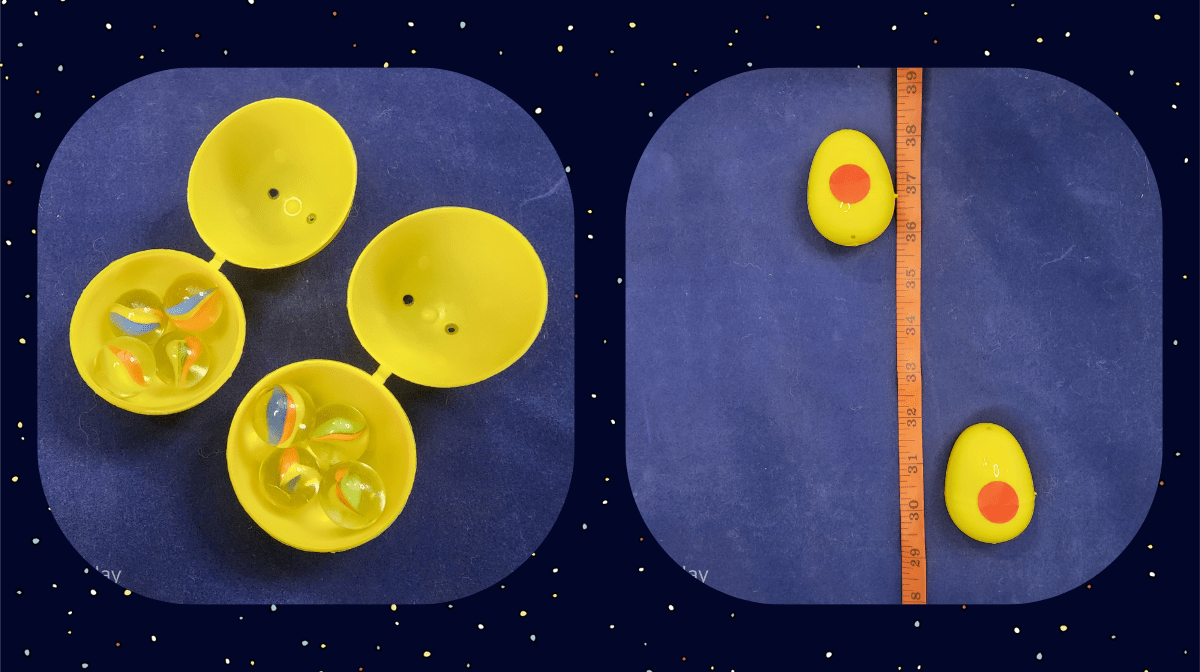
What happened? Did the eggs land at the same time, or at different times? How did the difference in distance the egg fell affect the landing? Was it what you expected?
Activity 2: Drop Objects Of Different Mass
- Assemble both plastic eggs. In one egg add 4 marbles. In the other, add no marbles.
- Hold both eggs, with the pointy end facing up, at 3 feet above the floor.
- Count to three and drop the eggs at the same time. Listen and watch carefully to see when they land in the bin. Record your results.
- Repeat the experiment several times.
- Try dropping the eggs from higher and higher distances, always dropping the two eggs from the same height and at the exact same time.
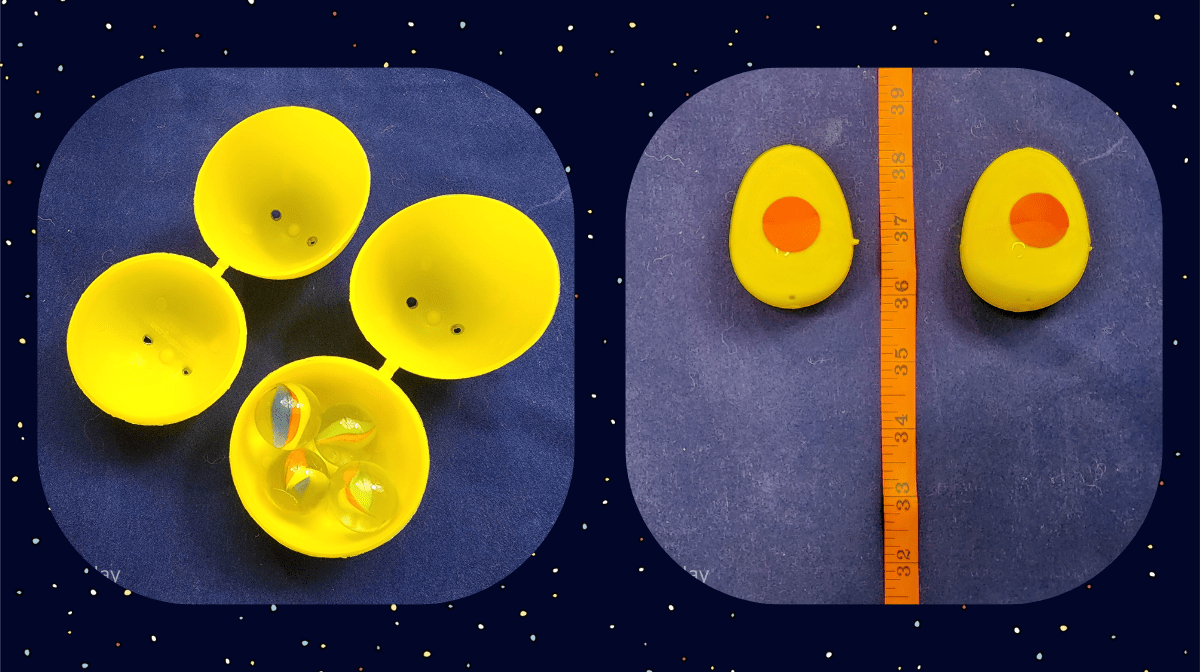
If desired, continue the experiment by varying the weight in one egg while leaving the other empty. Remember to change only one thing at a time, so that you know what change made a difference, if any. Be sure to record your results!
What happened this time? Was it what you expected? How did the results differ from the first activity?
You may feel confused or surprised right now. That’s ok! Many scientists throughout time have been confused by these results. So what’s going here?
Understanding Gravity In Free Fall
The eggs go from not moving at all to moving quickly. When they are dropped like this, they are in free fall. They accelerate as a result of the force of gravity on them. Acceleration is a change in velocity (speed) over time. Think of when you’re in the car and the driver pushes on the gas pedal. You suddenly accelerate until you reach the velocity at which the driver wants to travel, and then they stop accelerating, and maintain their speed. Our eggs are also accelerating, but their rate of acceleration is constant. They accelerate at a constant rate, dictated by Earth’s gravitational pull. For all objects on Earth, gravity accelerates them at a rate of 9.75 meters per second per second (32 feet per second squared!) If you drop an object from high enough, it will keep accelerating until the force of air resistance is sufficient to counteract the force of gravity, a speed called terminal velocity, which is different for differently shaped objects.
So the Earth’s gravity is pulling both eggs towards its center, but as you learned, mass affects gravity. But both the eggs have different masses, right? Won’t the heavier egg accelerate a little faster–and land first–because it is more massive?
Not quite. There is another force at play. Inertia.
Imagine that you’re kicking a resting, unmoving soccer ball. It isn’t terribly massive, so you’re able to kick it easily, accelerate it, and move it down field. Now imagine you had to kick a bowling ball instead of that soccer ball. Bowling balls are much more massive than soccer balls. It would take a lot more force to get it moving, wouldn’t it? That’s inertia, the tendency of objects to stay at rest or in motion. More mass, more inertia.
Objects with more mass have more inertia. So it takes more force to get the heavier ball moving. And that’s what happening with our eggs. Sure the heavier egg may be attracted to the Earth a little more due to gravity and have more weight, but it also takes a little more force to get it moving. The gravitational pull on the egg, and the egg’s own inertia cancel each other out.
Which means you’re left with the constant velocity from the pull of gravity. Both eggs land at the same time, regardless of the mass. This balance of gravity and inertia is really important to how the planets move. These two forces create our solar system orbits.
So what about the change in drop height? So long as the distance is sufficiently different, you will see the egg traveling the shortest distance hit the ground first. The eggs accelerate at the same rate and therefore match one another in speed at any given point in time on their descent. The only difference is that one egg has a shorter journey to the bottom. The less distance the egg needs to travel, the less time it takes.
In space, speed matters. The closer an object is to the Sun the faster it must be moving to maintain a stable orbit. That’s even more important to planets closer to the Sun, because those planets are more affected by the Sun’s gravity. So a planet like Mercury, which is close to the Sun and not very massive, must be moving quickly to avoid being pulled into the Sun. Jupiter on the other hand is much, much heavier and much further away, so it can move more slowly. You can experiment with model orbits using this online Gravity and Orbits Simulator from PhET Interactive.
Here are some questions to think about.
- How might these experiments relate to the constant pull of our massive Sun on the planets?
- How does the mass of different planets affect their inertia?
- What might these experiments tell you about how gravity works on Earth?
- What other experiments might you conduct to gain a better understanding?
Model Circular Motion And Orbits
Every planet in the solar system is affected by multiple forces. The gravity of the Sun pulls planets toward the center of the solar system. The inertia from the creation of the planets sent them flying in a straight line, perpendicular to the force of the Sun’s gravity. When these forces combine, they result in centripetal forces that push our planets in their circular motion around the Sun.
You will use a marble and a disposable plate to observe, examine, and test the limits of centripetal force.
Activity 1: Modeling Circular Motion
- Take a plate with a rim and put one marble on the plate, next to the rim.
- Give the marble a little push so that it travels along the inside of the rim Observe the movement.
- Repeat this several times, pushing the marble with different amounts of force.
What happens? How does the marble move when it encounters the rim? How does the force you apply to the marble affect how fast the marble moves? Think about what forces are causing the movement–what forces are acting on the marble, pushing it or pulling it, in addition to the force you applied with your finger? How does this model a planet in orbit around the Sun?
If desired, look around the house and try different sizes of plates. Does the marble need more or less of a push to make it around a larger plate? You can also experiment by using different sizes and weights of balls. Does a ping-ping ball or golf ball need more or less of a push? What about a bead or a dried pea? Experiment with different round objects and plates and record your observations.
Next, you’ll create a gap in the plate and test what happens when the rolling marble reaches that gap. Make a prediction: What will happen to the motion of the marble when it gets to the gap?
Activity 2: Inertia Takes Over
- Using a pair of scissors, cut a triangular wedge out of the plate, about two inches wide at the widest part on the edge of the plate and with the point of the triangle in the center of the plate.
- Put the marble on the plate, next to the rim, and give it a push along the rim away from the cutout gap. Observe the movement.
- Repeat this several times, pushing the marble with different amounts of force.
NOTE: You may want to wait to watch this video until after you have tried it yourself. Music by Toby Tranter.
What happens to the marble when it gets to the cutout part? Why do you think that happens? What force is affecting the marble?
The rim of the plate is keeping your marble from flying off into space. It’s acting like the Sun’s gravitational force, pulling the planets towards the center, perpendicular to the direction of the original force (your nudge). In this model, your finger is applying force in the way the original collisions of planets did during the formation of our solar system.
If there was not a Sun in the center of our solar system, what would happen? Would there even be a solar system anymore?
Model Circular Motion And Satellites
You’ve explored how gravity, inertia, centripetal force, and circular motion work. You’ve thought about how those forces relate to the motion of planets, dwarf planets, moons, and asteroids in our solar system. These natural satellites–objects that orbit other objects in space–have been circling the Sun for billions of years, set in motion, most likely, by the explosion of a star.
Humans have used their observations of the motion of planets to create their own satellites. Using advanced math and many calculations, scientists are able to launch spacecraft above the Earth and send them into orbit around our planet using centripetal force. In each case, scientists need to consider the effects of gravity and all the other forces on human-made devices so that they can control their motion as they move through space.
In this last activity, you’ll explore centripetal force and circular motion as you release your own satellite.
Activity
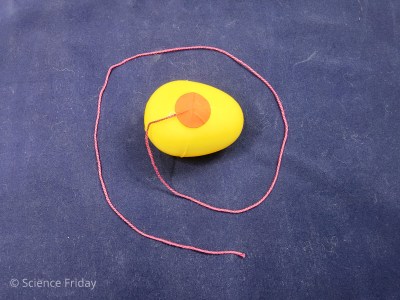
NOTE: This activity is best performed outdoors in a wide-open area. Make sure there are no people, cars, or other breakable things nearby.
- Cut a piece of string up to 2 feet long, depending on the amount of space you have to work. Tie a knot in one end of the string.
- Place one end of the string in between the two parts of an empty egg. Close the egg on the end of the string, trapping the string between the two sides of the egg. Use tape or stickers to securely close the egg and attach the string, if needed.
- Check that there is no one standing nearby one more time.
- Spin the string with the egg attached, creating a circular motion above your head. Observe the motion. Feel the tug of the string.
- Predict where the egg will fly off if you let the string go. Test your prediction.
- Repeat the above, but vary where in the circle you let go of the egg. You’ll want to spin the egg as slowly as possible so that you can actually determine the proper release point and see how it affects the flight.
- Repeat the experiment but change your spin in some manner. Try spinning it horizontally, vertically, and at different angles. Or reverse the direction of the spin, testing both clockwise and counter-clockwise.

How did your motion affect the movement of the egg when it was released? Did the egg move in different directions based on your movement? Did the speed of the spinning affect the movement? What trends or patterns did you notice? What do you think would happen if you placed a marble or two inside the egg?
In this final experiment, you were the Sun, but instead of gravity pulling Planet Egg into the center of your solar system, it’s the string. If you were the Earth, what natural satellite would the egg represent instead? If the egg was an artificial satellite orbiting the Earth, what would happen if it ran out of energy to push it forward?
Educators’ Note
The above activities work well as stations with groups of 3-5 students. The Pull of Gravity activities can be easily divided into separate stations. To simplify the preparation and save time, prepare and label eggs of different weights and attach a tape measure to the wall in advance. The Testing Circular Motion activities can be kept together as one station or separated. If you separate them, consider offering multiple sizes of plates and balls with the first activity and pre-cut several different plates with differently-sized gaps for the second. Students should always complete the first Testing Circular Motion activity before doing the second. For Send It Flying, you may want to use the activity as a closing demonstration and discussion.
Use What You’ve Learned
Now that you’ve explored gravity and centripetal force, use your knowledge to create a model satellite. Take a look at the design challenges below. Which problem would you like to solve? Using a plastic egg as the body, the marbles as weights, and other materials from around the house, adapt the egg to be a spacecaft. Use clay to make it more round. Add arms, solar panels, radio antenna, and other features if you like. Then test and adapt it until you can meet the challenge.
Challenge 1: Landing A Shuttle
When space shuttles return to Earth, scientists use their knowledge to make sure they land in a safe place. Place your bin from the first experiment somewhere in the room. Attach your spacecraft egg to the string. Can you launch your egg so that it lands in the bin? How must you spin it to land your egg safely every time?
Challenge 2: Slow The Fall
When shuttles and other objects return from space, gravity makes them accelerate very quickly as they approach the ground. They fall so fast that they can actually burn up due to friction with the air in our atmosphere. Re-entry can be very dangerous. How can you slow that fall for your spacecraft? Do some research on how space shuttles use air resistance to assist their return to Earth. Design a system to slow the landing of your spacecraft and test your ideas.
Challenge 3: Launch A Rocket
To launch satellites, rockets, shuttles and probes off the ground, scientists rely on thrust. Thrust works against gravity to lift our space vehicles. Can you devise a way to launch your satellite into the air?
We’d love to see your solutions! Share photos of your project with the hashtag #SciFriSunCamp or tag @SciFri on social media. Or send them directly to Science Friday using the Sun Camp photo submission form.
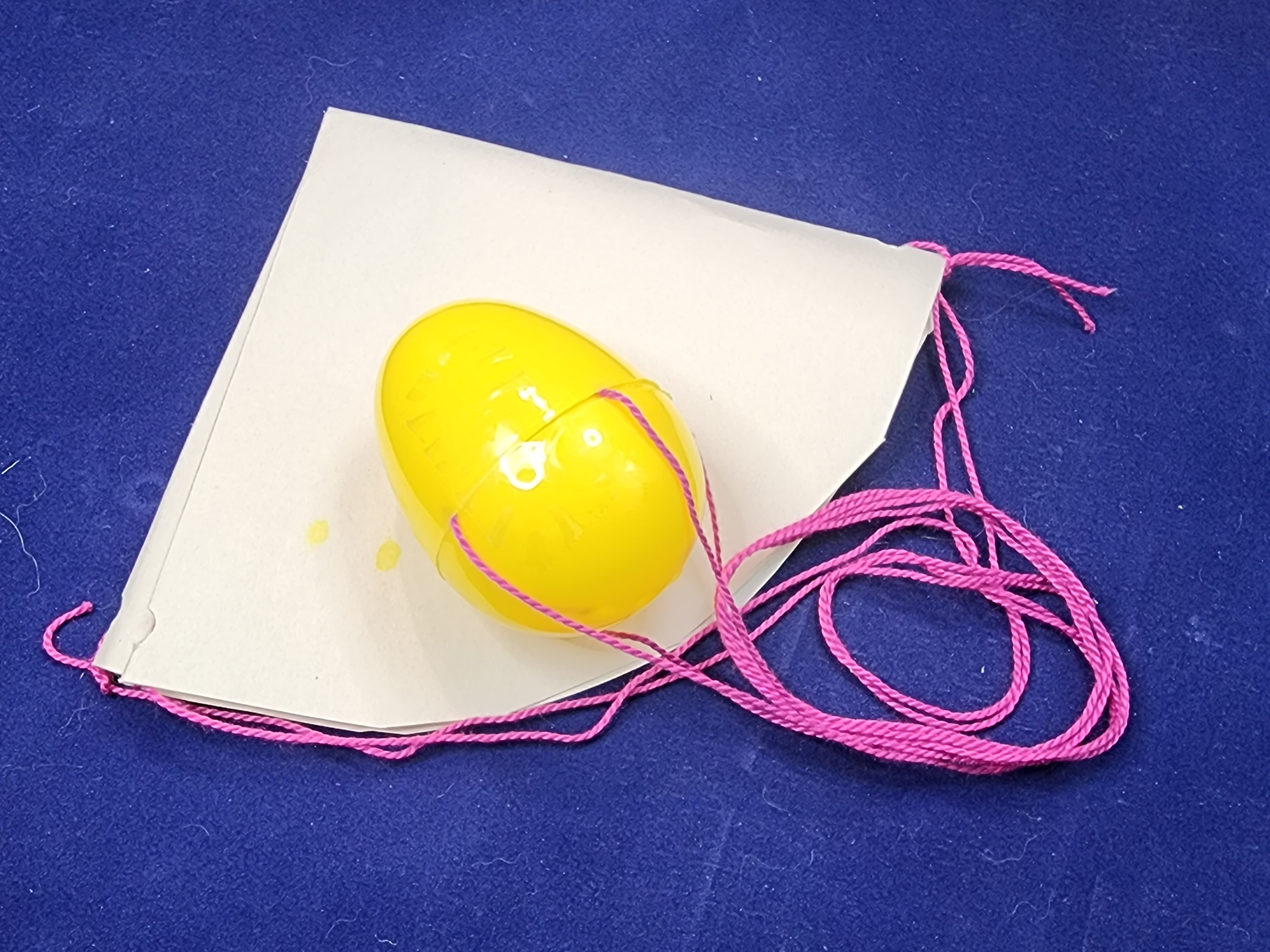
Keep Learning and Sharing
You can also read to learn more about the Sun and the solar system. Borrow The Magic School Bus Lost in the Solar System by Joanna Cole, My First Book of Planets by Bruce Betts, or Our Solar System: An Exploration of Planets, Moons, Asteroids, and Other Mysteries of Space by Lisa Reichley from your local library.
If you discover a great book, make sure you share your recommendation with Science Friday. We’d love to see your satellite models too. Share photos of your project with the hashtag #SciFriSunCamp on social media or send them to educate@sciencefriday.com.
NGSS Standards
- K-PS2-1: Plan and conduct an investigation to compare the effects of different strengths or different directions of pushes and pulls on the motion of an object.
- K-PS2-2: Analyze data to determine if a design solution works as intended to change the speed or direction of an object with a push or a pull.
- 1-ESS1-1: Use observations of the sun, moon, and stars to describe patterns that can be predicted.
- 3-PS2-1: Plan and conduct an investigation to provide evidence of the effects of balanced and unbalanced forces on the motion of an object.
- 3-PS2-2: Make observations and/or measurements of an object’s motion to provide evidence that a pattern can be used to predict future motion.
- K-2-ETS1-1: Ask questions, make observations, and gather information about a situation people want to change to define a simple problem that can be solved through the development of a new or improved object or tool.
- K-2-ETS1-2: Develop a simple sketch, drawing, or physical model to illustrate how the shape of an object helps it function as needed to solve a given problem.
- K-2-ETS1-3: Analyze data from tests of two objects designed to solve the same problem to compare the strengths and weaknesses of how each performs.
- 3-5-ETS1-1: Define a simple design problem reflecting a need or a want that includes specified criteria for success and constraints on materials, time, or cost.
- 3-5-ETS1-2:Generate and compare multiple possible solutions to a problem based on how well each is likely to meet the criteria and constraints of the problem.
- 3-5-ETS1-3:Plan and carry out fair tests in which variables are controlled and failure points are considered to identify aspects of a model or prototype that can be improved.
This activity is supported by the NASA Heliophysics Activation Team (NASA HEAT), part of NASA’s Science Activation portfolio.
Credits:
Written by Sandy Roberts.
Edited by Ariel Zych.
Illustration by Carrie Lapolla.
Digital Production by Sandy Roberts.
Have you registered for Sun Camp yet? Join today! You’ll get a weekly newsletter, access to interactive Q&As with real scientists, and extra resources for learning.
Meet the Writer
About Sandy Roberts
@KaleidoscopeSciSandy Roberts is Science Friday’s Education Program Manager, where she creates learning resources and experiences to advance STEM equity in all learning environments. Lately, she’s been playing with origami circuits and trying to perfect a gluten-free sourdough recipe.